Science Background
Freshwater and Heat: The Arctic freshwater cycle is a longstanding
framework for efforts to quantify and understand Arctic change due to
its important role in modulating the Arctic energy balance and,
further afield, global climate (e.g. Prowse et al., 2015;
Carmack et al., 2016). Freshwater
enters the Arctic upper ocean primarily through river discharge, Bering Strait inflow and net precipitation, with the
majority exiting about equally though the Canadian Arctic Archipelago
(CAA) and Fram Strait (Serreze et al., 2006; Haine et al.,
2015). Because salinity controls Arctic Ocean stratification, this
freshwater creates a cold, buoyant layer below the ice-ocean interface
that insulates the surface from the warmer, more saline Atlantic
waters below, thus modulating sea ice formation and melt and, through
this, coupling between the upper ocean and local atmospheric
forcing. Freshwater and heat exchange between the Arctic and North
Atlantic provide critical mechanisms through which the Arctic and
global climate interact. Arctic freshwater discharges through Davis
and Fram straits near deepwater formation regions west and east of
Greenland, where its buoyancy may act to modulate convective
overturning and deepwater formation (e.g., Karcher et al., 2005; Jahn
and Holland, 2013, Yang et al., 2016). Changes in Arctic freshwater
outflow also modulate the extent and strength of the North Atlantic
subpolar gyre, which can have profound impacts on fisheries (Hátún et
al., 2009), nutrient flux (Hátún et al., 2017) and on carbon uptake
and storage (Schuster and Watson, 2007) in this highly productive
region. Additionally, northward penetration of warm Atlantic waters
along the Greenland coast may accelerate the melting of marine
terminating glaciers (e.g., Holland et al., 2008, Straneo and
Heimbach, 2013, Myers and Ribergaard 2013, Gladish et al., 2014),
injecting additional freshwater into the system and contributing to
sea level rise.
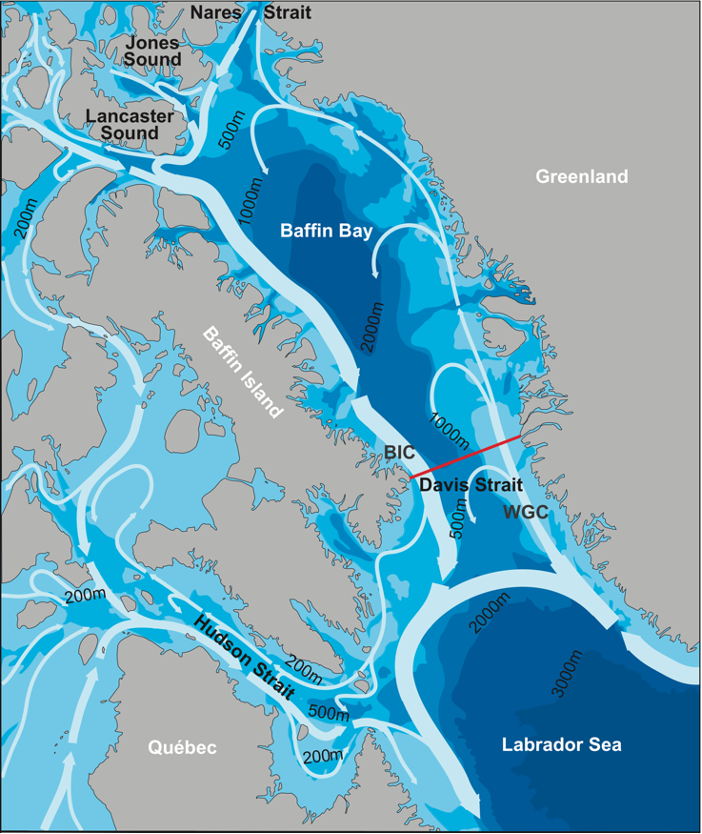
General circulation in Baffin Bay and Davis Strait (white arrows) and
the location of the 2004–10 moored array (red line). AW, by way of the
CAA, leaves Davis Strait as the broad, surface-intensified
BIC. Northward flow on the eastern side of Davis Strait consists of
the fresh WGC of Arctic origin on the shelf and warm, salty WGSC of
North Atlantic origin on the slope Curry et al., 2014.
Arctic Gateway: Davis Strait provides a single site for quantifying
both CAA outflow and northward fluxes along the West Greenland slope
and shelf that may impact land ice melt. The CAA component of Arctic
outflow enters Baffin Bay though four distinct passages (Bellot
Strait, Barrow Strait, Hell Gate/Cardigan Strait, and Nares Strait),
undergoing numerous transformations along its transit to Davis
Strait. By the time they reach Davis Strait, Arctic waters already
embody most of the transformation they undergo prior to exerting their
influence on the deepwater formation sites in the Labrador Sea. This
makes the Strait an ideal site to quantify the variability and
structure of the integrated CAA freshwater flux after it has undergone
these complex transformations, and just prior to entering the Labrador
Sea. Sustained observations at Davis Strait also provide early
detection of corrosive Arctic outflow into the subpolar North
Atlantic, where it may impact highly productive regions and important
commercial fisheries and document changes in these chemical states and
the marine ecosystem response to ocean acidification (Azetsu-Scott et
al., 2010;
Hammill et al., 2018).
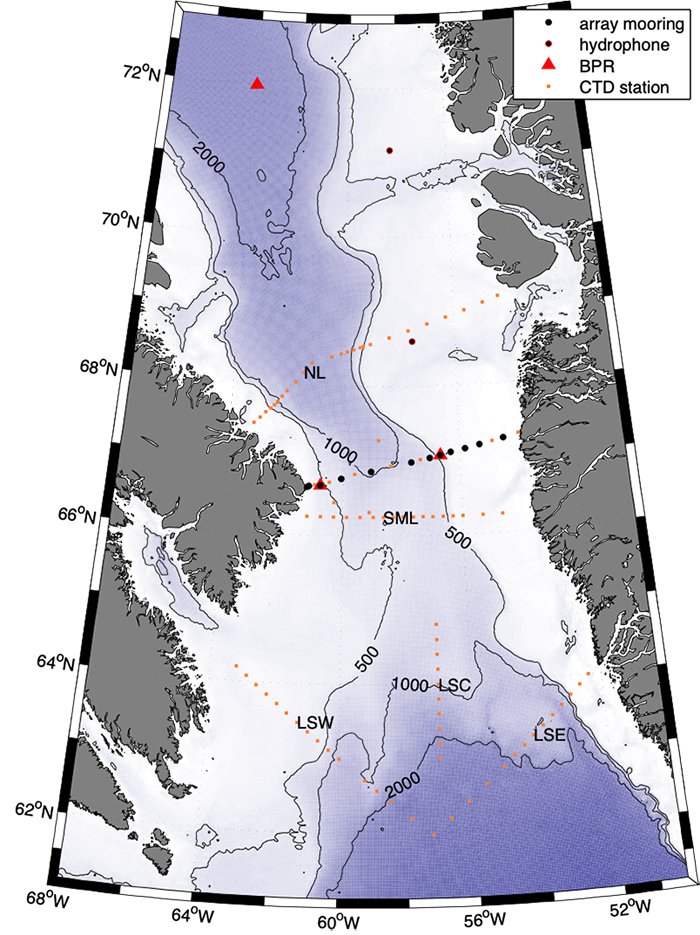
The Davis Strait observing system. Black dots mark mooring sites, red
dots mark hydirgraphic sampling stations and red tiangles indicate the
poistions of bottom pressure sensors (2020 and beyond).
Project Overview
The Davis Strait observing system was established in 2004 to advance
understanding of the role of Arctic – sub-Arctic interactions in the
climate system by collecting sustained measurements of physical,
chemical and biological variability at one of the primary gateways
that connect the Arctic and subpolar oceans. Efforts began as a
collaboration between researchers at the University of Washington’s
Applied Physics Laboratory and the Canadian Department of Fisheries
and Ocean’s Bedford Institute of Oceanography, but has grown to
include researchers from the Greenland Institute of Natural Resources,
Greenland Climate Institute, Danish Technological University,
University of Alberta and University of Colorado, Boulder. The project
is a component of the NSF Arctic Observing and Atlantic Meridional
Overturning Networks, and the international Arctic-Subarctic Ocean
Flux (ASOF) program, Global Ocean Ship-Based Hydrographic Investigations Program
(GO-SHIP), Global Ocean Acidification Observing Network
(GOA-ON), Arctic Monitoring Assessment Programme
(AMAP) and
OceanSITES system.
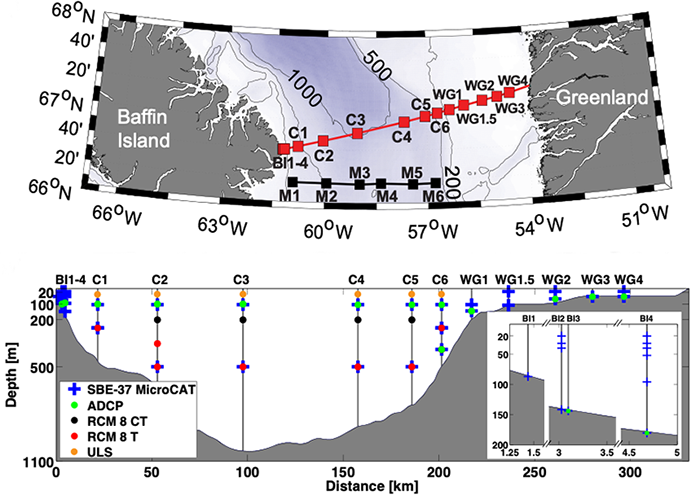
(top) Davis Strait 2004–10 (red line) and 1987–90 (black line) moored
arrays with squares indicating mooring locations. (bottom) Summary of Davis
Strait 2004–10 moored array instrumentation. Blue crosses indicate
SBE37 MicroCAT conductivity, temperature, and pressure recorders;
green dots represent RDI ADCPs; black dots denote Aanderaa RCM8
velocity, conductivity, and temperature recorders; red dots denote
Aanderaa RCM8 velocity and temperature recorders; and orange dots
denote ULSs. Inset image shows a close-up of the Baffin Island shelf
instruments. Spatial coverage varies from year to year throughout the
program. Curry et al., 2014
The initial system employed a mooring array measuring temperature, salinity, velocity, ice draft and passive acoustics (for marine mammal presence), annual hydrographic sampling for chemical properties and year-round sampling using autonomous, long-endurance Seagliders. The program included development of new technologies to enable year-round measurements in ice-covered waters, specifically Seagliders with enhanced autonomy and acoustic navigation and an inexpensive, light-weight approach for collecting moored measurements near the ice-ocean interface. To decrease cost and thereby facilitate sustained operations, in recent years the measurement program has consolidated to rely solely on the mooring array and biennial chemical and bioloigical sampling, with the Seaglider component ending in 2014. Launched in 2020, the current system has expanded to include biological measurements across multiple trophic levels (phytoplankton to marine mammals) and numerical modeling at both regional and climate scales.
Highlights
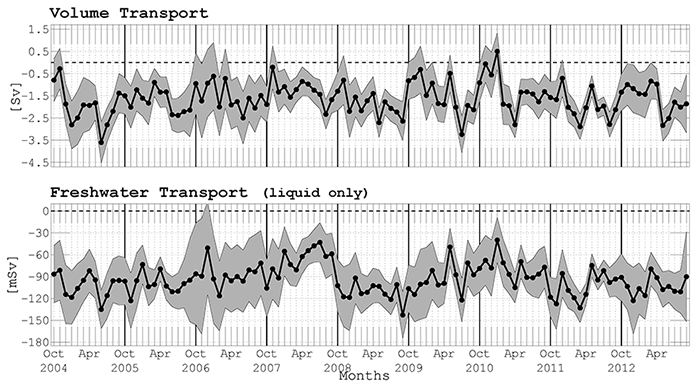
Monthly volume and freshwater (relative to S = 34.8) between October
2004 and September 2012, calculated from objectively analyzed velocity
and salinity fields, integrated from 640 m (sill depth) to the sea
surface. Negative values indicate southward transport, with grey
shading marking uncertainties in the estimates Curry et al.,
2014.
Year-long sampling of Davis Strait by Seagliders. The background chart
displays sea ice concentration from SMMR and SMM/I (National Snow and
Ice Data Center and NASA Goddard Space Flight Center), with blue
marking open water and white indicating full ice cover. Green symbols
mark mooring locations. Gliders operating under ice cover geolocated using
the array of acoustic navigation sources (moorings with green circles).
Moving yellow, red and magenta symbols mark glider positions from three
successive glider missions, with a 7-day tail. From 9 June 2010 - 17 Jun
2011, gliders occupied 34 repeat sections, with 7 months operating in
partial or full ice cover.